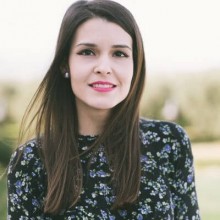
Dr. Demeridou is currently a postdoctoral fellow, her current research interests include two Dimensional Materials (2D), μ-Photoluminescence Spectroscopy (μ-PL), Raman Spectroscopy, Pulsed laser irradiation of the atomically thin lattice in a precursor gas atmosphere. Her research interests, also, lie in the area of Spin-Valley Polarization measurements in TMDs and the effect of carrier doping.She obtained her M.Sc. in master program “Microelectronics-Optoelectronics” in Physics from University of Crete, Greece in 2016. In 2014, she joined the “Ultrafast Laser Micro and Nano Processing” Group of the IESL, FORTH. She carried out her master thesis: “Photochemical Doping of mechanically exfoliated monolayer WS2”.She received her Bachelor degree in Physics from University of Crete, Greece in 2013.
Education
- 2021, PhD, Department of Physics, University of Crete, Greece.
- 2016, M.Sc, Department of Physics, University of Crete, Greece.
- 2013, B.Sc. Department of Physics, University of Crete, Greece.
Career
- February 2019 – May 2019, Teaching Assistant as a PhD student, at the postgraduate course “Advanced Photonic characterization of materials for energy applications”, Master Program: Nanotechnology for Energy Applications, Institute of Electronic Structu
- October 2017 – May 2017, Teaching Assistant as a PhD student, at the undergraduate course “Advanced Physics Laboratory”, Physics Department, University of Crete. Instructor: Prof. Eleftherios Iliopoulos.
- October 2013 – May 2014, Teaching Assistant as a Master student, at the undergraduate course “Advanced Physics Laboratory”, Physics Department, University of Crete. Instructor: Prof. George Kiriakidis, Prof. Eleftherios Iliopoulos.
- February 2013 – May 2013, Teaching Assistant as a graduate student, at the undergraduate course “Physics Laboratory III: Optics”, Physics Department, University of Crete. Instructor: Prof. Petros Rakintzis.
- October 2012 – December 2012, Practical Teaching at the Experimental General Lyceum of Heraklion, at the undergraduate course “Teaching Physics I”, Physics Department, University of Crete. Instructor: Prof. Ilias Perakis.
Interests
- Mechanical Exfoliation of Two Dimensional Crystals
- Pulsed laser irradiation in a precursor gas atmosphere
- Raman Spectroscopy
- Micro-Photoluminescence Spectroscopy
- Micro-Differential Reflectance Spectroscopy
- Spin Valley Polarization measurements
- Cryogenic System
Ms. Lefki Chaniotaki is a master student at the department of Materials Science and Technology at the University of Crete. She has received her bachelor in Materials science and Technology in 2019. She joined the Ultrafast Laser Micro- and Nano- Processing Laboratory of IESL in 2017, where she obtained her internship with Evi Kavatzikidou. She made positive PLGA replicas of silicon microstructures via soft lithography and then characterize them with UV-VIS, SEM and RAMAN. Moreover, in 2018-2019 she did her bachelor’s thesis in the same group, but she combined the prosthetic technique (FFF 3D printer) and ablative technique (laser ablation) to make 3D scaffolds with 3D ablation. Finally, in 2020 she started her master thesis where she continues the same topic as her bachelor’s thesis.
Education
- 2019-present, master in Material Science and technology, University of Crete, Greece.
- 2014-2019, Bachelor in Materials Science and Technology, University of Crete, Greece.
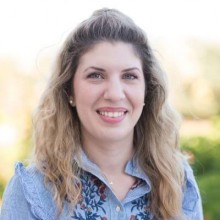
Dr. Eleftheria Babaliari is a Postdoctoral Researcher at the
Foundation for Research and Technology - Hellas (FORTH), Institute of
Electronic Structure and Laser (IESL). She received a Bachelor’s, a
Master’s and a Ph.D.’s Degree in Materials Science and Technology from
the University of Crete, Greece in 2012, 2015 and 2020, respectively.
During the undergraduate program, she joined the Polymer Group of the
IESL, FORTH where she worked on the rheology of hard spheres colloidal
suspensions and binary mixtures. Ιn the master’s degree program, she
joined the Biomaterials Lab of the Department of Materials Science and
Technology in Crete where she studied the effect of microfluidics in
biomaterials for bone tissue engineering applications. During her
Ph.D., she joined in 2015 the Ultrafast Laser Micro and Nano
Processing Group of the IESL, FORTH performing research on the laser
microstructured scaffolds for tissue engineering under dynamic cell
culture conditions. Her current research interests include the
development of laser-microstructured scaffolds for tissue engineering
applications, using ultrafast laser structuring, and the study of the
combined effect of flow-induced shear stress and surface topography on
tissue growth.
Education
- 2015 – 2020, Doctor of Philosophy, Materials Science, University of Greece, Crete
- 2012 - 2015, Master of Science, Materials Science, University of Crete, Greece
- 2008 - 2012, Bachelor, Materials Science, University of Crete, Greece
Career
- 01/2021 – present, Postdoctoral Researcher at Foundation for Research and Technology – Hellas (FORTH), Institute of Electronic Structure & Laser , Heraklion, Crete, Greece
- 01/2023 - 12/2023, Assistant in the laboratory of laser and modern physics, University of Crete, Greece
- 09/2022 - 12/2022, Assistant in the laboratory of laser and modern physics, University of Crete, Greece
- 01/2021 – 12/2021, Assistant in the laboratory of laser and modern physics, University of Crete, Greece
- 01/2020 – 12/2020, Assistant in the laboratory of laser and modern physics, University of Crete, Greece
- 12/2015 – 10/2020, PhD thesis: Laser microstructured scaffolds for tissue engineering under dynamic cell culture conditions, Foundation for Research and Technology – Hellas (FORTH), Institute of Electronic Structure & Laser , Heraklion, Crete, Greece
- 11/2019 – 12/2019, Assistant in soft matter lab, Department of Materials Science and Technology, University of Crete, Greece
- 01/2019 – 12/2019, Assistant in the laboratory of laser and modern physics, University of Crete, Greece
- 11/2018 – 12/2018, Assistant in soft matter lab, Department of Materials Science and Technology, University of Crete, Greece
- 01/2018 – 12/2018, Assistant in the laboratory of laser and modern physics, University of Crete, Greece
- 11/2017 – 12/2017, Assistant in soft matter lab, Department of Materials Science and Technology, University of Crete, Greece
- 01/2017 – 12/2017, Assistant in the laboratory of laser and modern physics, University of Crete, Greece
- 09/2016 – 12/2016, Teaching Assistant in chemistry lesson, Department of Materials Science and Technology, University of Crete, Greece
- 09/2015 – 12/2015, Teaching Assistant in chemistry lesson, Department of Materials Science and Technology, University of Crete, Greece
- 02/2014 – 04/2015, Master thesis: Microfluidics in biomaterials for bone tissue engineering applications, Foundation for Research and Technology - Hellas (FORTH), Institute of Electronic Structure & Laser – Biomaterials Lab (University of Crete)
- 09/2014 – 12/2014, Teaching Assistant in chemistry lesson, Department of Materials Science and Technology, University of Crete, Greece
- 10/2013 – 01/2014, Teaching Assistant in chemistry lesson, Department of Materials Science and Technology, University of Crete, Greece
- 09/2011 – 10/2012, Diploma thesis: Rheology of hard sphere colloidal suspensions and binary mixtures, Foundation for Research and Technology - Hellas (FORTH), Institute of Electronic Structure & Laser, Heraklion, Crete, Greece
- 2/2011 – 05/2011, Practical exercise: Rheology of hard sphere colloidal suspensions and binary mixtures, Foundation for Research and Technology - Hellas (FORTH), Institute of Electronic Structure & Laser, Heraklion, Crete, Greece
- 02/2011 – 05/2011, Assistant in chemistry lab, Department of Materials Science and Technology, University of Crete, Greece
- 09/2010 – 12/2010, Teaching Assistant in chemistry lesson, Department of Materials Science and Technology, University of Crete, Greece
Interests
- Microfluidics
- Laser Processing
- Tissue Engineering
- Biomaterials
- Polymers
Awards/Prizes/Distinctions
- Best Poster Presentation Award – IESL, «Combined effect of shear stress and topography on Schwann cells behavior E. Babaliari, P. Kavatzikidou, A. Mitraki, Y. Papaharilaou, A. Ranella, E. Stratakis» 12th FORTH Retreat, Patra, Greece, 15 – 16 October 2019
- 2017, Hellenic Foundation for Research and Technology (ELIDEK) (PhD Scholarship)
- 2016 – 2019, State Scholarships Foundation (IKY) (PhD Scholarship, «ΕSPΑ 2014 – 2020»)
- 2016, Onassis Scholarship, Onassis Foundation (PhD Scholarship)
- 2011 – 2012, State Scholarships Foundation (IKY) (Undergraduate Scholarship)
- 2011 – 2012, Soukouli Scholarship, University of Crete (Undergraduate Scholarship)
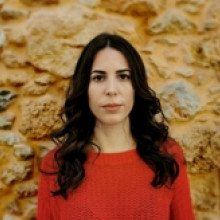
Α. Argyrou is a PhD student at the department of Chemistry of the University of Crete. She received her bachelor in Physics in 2017 and her master in Material Science and Technology in 2019. She joined the Ultrafast Laser Micro- and Nano- Processing Laboratory of IESL in 2018, where she obtained her master thesis. Her current research interests include the development and characterization of nanostructures for gas sensing applications.
Education
- 2019 - PhD student in Chemistry Department, University of Crete
- 2019 - Master in Materials Science and Technology (in progress) , University of Crete
- 2017 - Bachelor , Physics , University of Crete
Interests
- Synthesis of perovskite nanocrystals for gas sensing applications
- Optical and structural characterization of nanocrystals
- Synthesis and characterization of nanoparticulate films
- Elucidation of the microscopic physical mechanisms involving nanocrystal systems
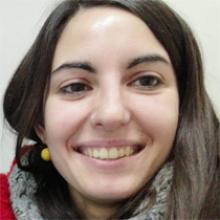
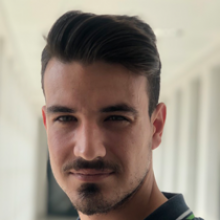
I am a physicist (UoC 2020) currently working on a MSc at the Department of Materials Science & Technology of the Universoty of Crete, carrying out the research work of it at the Dynamics Group of IESL-FORTH.
My research focuses on building a Time-Resolved Electron Diffractometer, investigating chrial molecules with Photoelectron Circular Dihroism and probing fuels with spectroscopy.
Education
- 2020 B.Sc. in Physics, Physics Dpt., University of Crete, GREECE
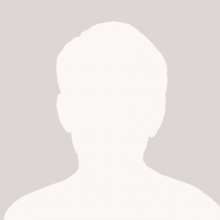
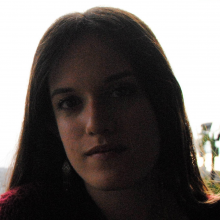